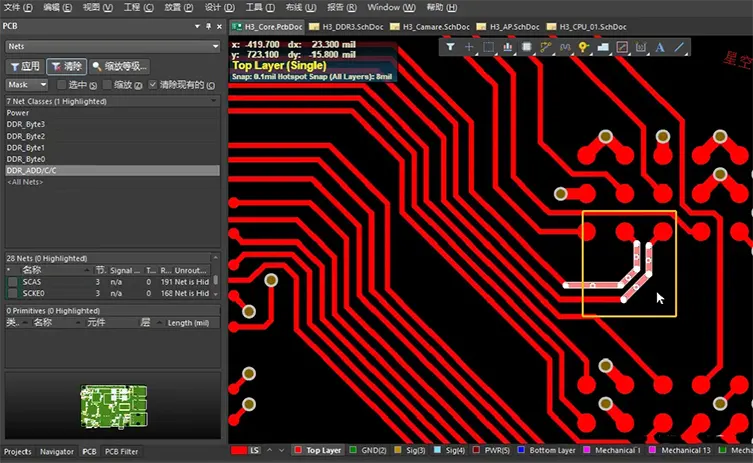
JarnisTech provides impedance control PCBs manufacturing. We accept designs with multiple impedances, precisely manufacturing control using a strip sample and a report on the impedance test, send your PCB design files to [email protected] and estimate the cost today!
What Is Impedance
Impedance is the combination of the capacitance and inductance of a circuit operating at a high frequency that is expressed in Ohms. Every material has some degree of resistance to current flow. When voltage and current change, resistance results from capacitance and inductance interacting with each other. In DC circuits, there isn’t any reactance, and copper conductors’ resistance is usually minimal.
What Is a Controlled Impedance of PCB
Controlled impedance PCBs include printed circuit boards that have controlled impedance throughout the process that involves designing, manufacturing and performance. It is difficult to regulate impedance without carefully planning the trace of your PCB and the working environment, as the impedance can vary from one point to the next within the trace. When frequencies are high PCB trace don’t behave as simple circuit connections. Controlled impedance allows us to ensure that signals aren’t affected by the route they take through the printed circuit board.
PCB controlled impedance refers to the matching of the properties of the PCB base material with trace dimensions and locations so that the impedance of a trace’s signal remains within the percentage of a specific value. Controlled impedance PCBs offer reproducible high-frequency performance.
How Can Create a Board That Has an Impedance That Is Controlled
Designing a controlled impedance PCB should the following controlled impedance routing tactics:
Identify Which Signals Require CI
In most cases, electrical engineers specify which signal nets require a specific controlled impedance..If they do not, the designer must check the integrated circuit’s datasheet to determine which signals require controlled impedance. Datasheets typically provide specific guidelines for each type of signal as well as their impedances. In the datasheets and application notes, you can also find information on spacing rules and which layer to route different signals. DDR trace, HDMI traces, Gigabit Ethernet tracks as well as RF signaling are a few examples of traces with controlled impedance.
Schematic Should Be Annotated With the Impedance Requirements
The creation of a board begins with the development of circuit schematics by the engineer who designed it. The engineer has to define controlled impedance signals within the schematic and then classify certain nets as either differential pairs (100Ω, 90Ω or 85Ω) and single-ended networks (40Ω, 50Ω, 55Ω, 60 Ω or 75Ω). It is a good practice to include an N or P polarity indicator following any net name of differential pair signals on the diagram. Engineers should also indicate specific controlled impedance layout design guidelines (if there are any) to be adhered to by the layout designer on the schematic or in a separate “Read My” file.
Determine the Trace Parameters to Control CI
The PCB trace can be determined by its thickness, width, and height along with the dielectric constant (Er) of the PCB material onto which the trace is etched. When designing PCBs that have controlled impedance, it is vital to be aware of these parameters. It is possible to provide the manufacturer along with layers count, the amount of the impedance lines on particular layers (50Ω, 100Ω on layer 3) and also the material to designing PCBs.
The manufacturer will provide you with a stack-up that lists the widths of the traces on each layer and how many layers as well as the thickness of every dielectric within the stack-up, the thickness of the trace and the PCB material. He also manages the requirements for controlled impedance by making a calculation of the practical thickness, width, and height of the traces that require impedance control. Follow the following rules to determine how impedance is dependent on the dimensions:
The impedance is proportional to the trace’s width and thickness.
The Impedance is proportional to the thickness of the laminate and is in inverse proportion in proportion to the square root dielectric constant (Er).
Factors That Affect Control of Impedance During PCB Design
Some factors that affect impedance control in PCB design are trace width and copper thickness, as well as dielectric thickness and dielectric constant. Here is a detailed description of the impact these variables have:
The width of the trace: On a PCB, the trace width is equal to the width of the copper foil plus its coating. As the trace width increases the impedance decreases. When the designers design specifications for PCBs, they base trace width on various factors, such as capacity and temperature increase. When they’ve got a specific impedance in mind, they could make a PCB to accommodate that.
The Copper Thickness
on PCB can affect the impedance too. Like increasing the width of the trace, as the copper thickness increases, the impedance will tend to decrease. If designers wish to reduce impedance, they could increase the weight and thickness of the copper. However, when they wish to permit higher impedance, they could reduce the weight of copper and thickness.
Dielectric Thickness
A dielectric thickness describes the thickness of the insulating material between traces in electronics. Impedance and dielectric thickness are in a logarithmic relation significant increases in thickness can produce modest increases in impedance. To decrease impedance, designers can significantly decrease the thickness of insulation material.
Dielectric Constant
A material’s dielectric constant is the ratio of its electric permittivity to a vacuum’s. In a PCB, The dielectric constant of a PCB varies inversely with frequency. When a PCB have a low, stable dielectric constant and it is suitable for high frequencies and controlled impedance. A more difficult dielectric constant may cause impedance to change in unexpected ways.
The general rule is that board designers along with electronic manufacturing service professionals should take into consideration a range of elements that may affect the performance of the product. This includes the control of impedance since it can significantly influence the performance and integrity of the signal on PCBs. Manufacturers can manage impedance by adjusting the PCB trace’s configuration as well as their dielectric constant for the PCB’s material. If your requirements regarding impedance are very specific, it is recommended to provide the desired impedance tolerance as well as value prior to the time of purchase. Speak to the PCB assembly specialists for further assistance in understanding how to control impedance.
PCB Transmission Line
Interconnections line that move signals from a PCB’s transmitters to its receivers, we call PCB transmission lines. Each transmission line is comprised of at minimum two conductors. And there must be at least one return path on every transmission line, and one conductor must serve as a signal trace. The majority of return paths are ground planes. On a PCB, dielectric material makes up the volume between conductors. The dielectric layer is used to separate signals from the ground plane as well as other conductors.
Transmission lines require impedance controls to resolve issues with signal integrity. Impedance control is crucial to transmitting signals as waves without distortion when high-frequency signals are transmitted through cables that are PCB-based. If the impedance of transmitters differs, then a signal reflection takes place. The reflected signals distort the original signal by superimposing on the original signal.
It is generally impossible for distorted signals to perform their intended functions. Implementing a uniformly controllable impedance at every point reduces distortions in signal. Impedance control improve signal integrity.
The PCB’s physical dimensions, as well as the materials contained within its dielectric layer determine the resistance of the circuit that can be measured as Ohms.
Transmission Lines for PCB Requiring Controlled Impedance
In addition to the distinction between stripline and microstrip lines, other differences separate various transmission lines. The process of embedding the trace that is exposed from microstrip lines in the dielectric coating is an example of creating an embedded microstrip. Another common modification is to join PCB transmission lines are frequently coupled into differential pairs.
A differential pair can be described as a well-balanced pair of conductors that function as transmission lines. They transfer power and can be subjected to the effects of impedance. Each of the below PCB transmission lines must be controlled in impedance control:
● Microstrips with a single-ended end.
● Microstrips that have differential pairs
● Embedded with microstrips
● Single-ended stripline
● Stripline with differential pairs
● Co-planar waveguides with single-ended ends
● Differential pair of co-planar waveguides
Fabrication for Impedance Control
After you design the perfect PCB, you can send it off to your CM confident that it will be implemented in accordance with your design intent. You alse can get help with impedance control from some manufacturers, but only if you provide them with the right information. At the same time, to ensure everything comes out within tolerance, you must still need to keep an eye on materials.
Many manufacturers let you perform one of two things either specify the dielectric material thickness, in addition to the width of copper or indicate your desired resistance. If you decide to perform all the calculations yourself, I’m sure that your CM will not be a snob. Be sure to perform the calculations correctly. In the event that you don’t, you can inform your CM to know the impedance you’re looking for and they’ll modify the substrate and trace to meet your desired number. Ensure nothing else is affected by their changes by double-checking it after they make their changes.
When you specifying materials, be aware that certain materials are available in sizes that are standard, and all inputs come with specific tolerances. For example, the dielectric constant for FR4 could be quite different in one batch. If you require something that’s more reliable, then you must look into higher-speed-specific materials. It is easier to alter the thickness of certain layers or substances than others. Make sure you talk to your CM and understand their strengths and limitations prior to you begin designing something that isn’t feasible to build.
With impedance control, long or high-speed traces will arrive intact with minimal distortion. The primary two knobs that you can use to accomplish these are the dielectric material, thickness, as well as width of copper and routing. The main goal for substrates is to make them as homogeneous as you can. For your traces, ensure your traces are routed over a solid plane at least on one side, avoid gaps, and keep differential pairs’ lengths similar.
Not least, it is necessary to design your PCB. Be sure to select a CM who will be able to be in contact with you throughout the entire process and offer useful suggestions. Jarnistech is the PCB industry leader in quickly designing custom boards and meeting the exact specifications of the design.
Controlled Impedance: Why Do You Need
In general, you’ll require controlled impedance on PCBs in high-speed digital programs, for example, RF communications, telecommunications, computing, and other applications that require signal frequencies over 100MHz, high-speed signal processing as well as high-quality analog videos such as DDR, HDMI, Gigabit Ethernet and more.
At high frequencies, the signal trace tracks on a PCB function as transmission lines that have impedance at every point of the trace of signal. If this impedance differs between one location and the next, then there will be reflections of the signal that’s magnitude depends on the differences in the impedances. The higher the difference then the more intense reflection will be. The reflection will be towards the opposing direction of the signal, meaning that the reflected signal will be superimposed onto the primary signal.
The signal that was originally sent will be altered as the signal to be transmitted from the transmitter side will have been altered after it has arrived on the receiving side. It is possible for the distortion to be so severe that the signal cannot carry out its intended function. In order to ensure undistorted signal transmission, PCB signal traces need to have a uniformly controlled impedance in order to reduce distortions due to reflections. In order to improve the integrity of the signals on the PCB traces, this is the first step.
Generally, uniform transmission lines on PCBs are uniformly spaced from return path conductors, the transmission lines have a definite width and height, usually a plane at a certain distance from the signal trace.
Other Reasons To Require A PCB For Impedance Control Include
More Power for Signals Required
PCB trace functions transmit a driver device’s signal energy to devices that can receive it. The power needs to be transmitted through all of the trace’s distance. However, the highest signal power can only be achieved using the PCB’s impedances that match.
This is the main reason why an impedance control PCB is required. It has an impedance match, which allows enough power from the device’s driver and reaches the receiver.
Enhanced Performance
If you’re in search of PCBs that guarantee the highest quality of performance for your device, then you should consider an impedance control PCB.
Poor PCB layout and design are the primary reasons for device failure when it comes to signal integrity and power. The layout stage in the production of PCB is often an important one. If the layout isn’t done properly, it is likely that there’ll be a degrading of high-speed signals that are propagated through the receiver.
It is common for devices controlled by impedance control PCBs to consume less energy and operate faster. This PCB type permits devices to operate more efficiently over a longer period of time, increasing their reliability in control and performance.
Control the Flow of Energy
It’s true that an impedance control PCB uses less energy. But, if you want to regulate your energy flow through your projects, then an ideal option is the Impedance Control PCB. It is true that control of impedance is important for transitioning from low to higher ohm settings in which there is impedance.
What is the reason for this? These changes could cause an energy reflection, which appears like strong and powerful pulses. These pulses could interfere with an energy flow. If your application is dealing using electronic devices that operate using high power, such as those used for RF applications, it is necessary to utilize an impedance control PCB.
Management of Electromagnetic Interference
If you’re using an impedance-control PCB, you don’t need to be concerned about disruptions to circuits, as a result of electromagnetic interference.
In concerns the PCB area, even a pulse of reflection energy can destroy circuits completely. The damage usually is extended to the neighboring components. Additionally, there is an opportunity to disrupt an energy flow, causing the operation of the product to fail.
Controlled Impedance: When To Use It
When a signal needs to have an impedance that is specific to it to function correctly, a controlled impedance must be utilized. In high-frequency applications, matching an impedance that is compatible with PCB trace is crucial to keeping the integrity of data and signal clarity. When the trace’s impedance PCB trace that connects two components is not in line with the component’s characteristic impedance, it could result in an increase in the switching time within the circuit or device. Also, there could be accidental mistakes.
The Calculation of Impedance Control
It is crucial to determine the impedances of a PCB. Whether is crucial and requires specialized methods for measuring. Let’s examine the impedance control methods that are reliable, precise and solid.
Smith Chart
The Smith chart was in use prior to the time computers and PCB design software became commonplace. The chart helps define the relationship between load impedance and source using graphic images. As well as relies on an array of mathematical calculations, it provides you with a trace’s impedance value.
Computing Simulation
Modern software uses sophisticated technology to calculate the impedance of a control. The advantage of this software is that they are able to integrate your design and design concerns into a model to show an almost finished product. But, this kind of software can be expensive to purchase and is used to design a final PCB design.
Online Impedance Calculator
Online calculators are more effective and widely used than design software using impedance control on PCBs. You can determine how to control the impedance of the trace you make using these calculators. These calculators aren’t exactly as accurate as simulators, but they do have a rough estimate to start the process. A few minor tweaks later can keep you in a secure zone as the majority of these calculators are based on standard numbers from the design guidelines of high-speed impedance boards.
The three methods above are a great base for your calculations. Whatever method you decide to use, Compared to not calculating impedance, you’ll start with a set of rules that are safe.
Application of Controlled Impedance
Controlled Impedance is a good option for PCBs that are used in digital applications, such as:
● Telecommunications
● Computing 100MHz or more
● High-Quality Analog Video
● Signal Processing
● RF Communication
Common Controlled Impedance Types
Single-ended Impedance
On PCBs, single-ended impedance is a common type of impedance. single-ended impedance is the impedance of the length of the trace. The CAM engineer at your PCB provider will first calculate the impedance controlled to determine how much traces alteration and the best way to alter the match of materials with the PCB’s stack-up. In the following step, the planner will arrange for the impedance-controlled PCB production to take place.
Measurement of single-ended impedance can be done on a single trace without coupling to the adjacent trace. Based on the Coated Microstrip interfaces in Polar SI9000, the single-ended impedance is influenced by the thickness of the PCB board substrate and dielectric value of the substrate trace’s upper and lower width and size (/copper weight) and the coating (/solder mask) thicknesses and the dielectric coating. The primary factors that determine the single-ended impedance are dielectric Er and the width of the trace. In addition, other factors are also not affected by the impedance calculation results.
Differential Impedance
In circuit board design and manufacturing, differential impedance is another type of impedance commonly used. Differential impedance refers to the difference in impedance between two traces, also known as differential pair traces when driven by opposing and equal to the polarity signal.
Based on the Edge-Coupled Coated Microstrip interfaces in Polar SI9000, we can see that the differential impedance is influenced by numerous factors, including PCB thickness and dielectric Er value trace widths as well as trace spacing (/spacing) and track thickness (/copper thickness) and the coating (/solder mask) thicknesses as well as the thickness of the dielectric. When dealing with differential impedance calculations, the most important factors in determining the impedance are the substrate size, Er value, the width of the trace and its spacing.
Both Single-ended Impedance, as well as Differential Impedance can be added to the impedance that is used to hatch PCBs with flex and rigid-flex.
Conclusion
We hope that you’ve been able to get answers to any questions that have been that are circulating in your head regarding Impedance Control PCB. As we mentioned before, understanding impedance, and also the issues related to impedance control, isn’t as simple.
We have tried our best to present the subject in depth and, in the end, I think you’ve become an expert in the impedance control PCB.
The circuit’s impedance is determined by its physical dimension (line wide/space) as well as the material used in the circuit. It is expressed as Ohms (Ω).
Controlling PCB impedance is one of the most important concerns and difficult issues encountered when it comes to high-speed PCB development. If you are an impedance-control engineer, you need to know what influences PCB impedance as well as how to calculate the impedance.