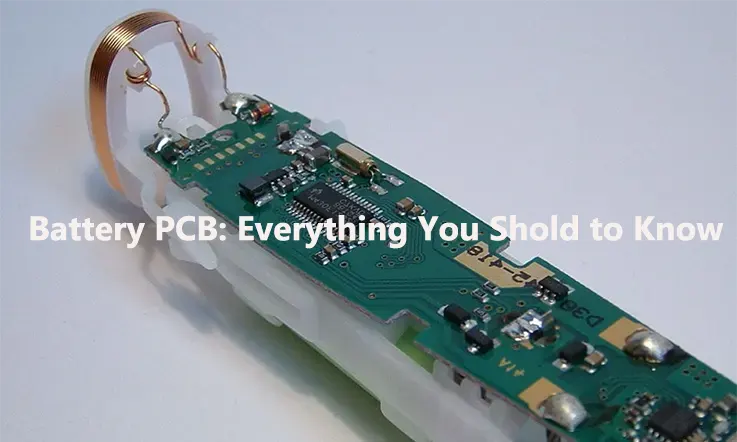
Battery PCBs, also known as printed circuit boards, are essential components found in a wide range of energy-powered devices. They play a crucial role in the functioning of various everyday electronics.
In China, JarnisTech holds a prominent position as the leading company specializing in Battery PCBs. As a result, we frequently receive inquiries from our valued customers seeking information about battery PCBs. Regrettably, there is a limited amount of readily available information on this topic online. Consequently, the purpose of this FAQ is to provide clear and concise answers to address any queries or concerns regarding battery PCBs, allowing for a better understanding of their significance and application.
Introduction to Battery PCBs
Battery PCBs, also known as battery protection circuit boards, are electronic circuits designed to protect rechargeable batteries from damage due to overcharging, over-discharging, short-circuits, and other potential hazards.
These circuits are typically integrated into the battery pack and provide safety features that help to extend the battery’s lifespan and prevent accidents. Battery PCBs are becoming increasingly important as the use of rechargeable batteries continues to grow in various applications, including consumer electronics devices, electric vehicles, and energy storage systems.
There are different types of battery PCBs available in the market, including simple protection circuits that provide overcharge and over-discharge protection, and more advanced battery management systems (BMS) that offer additional features such as balancing the charge across multiple cells in a battery pack. The choice of the type of battery PCB depends on the specific application and the requirements of the battery pack.
All in all, battery PCBs play a critical role in ensuring the safety and longevity of rechargeable batteries, and their importance is expected to continue to grow as the use of these batteries continues to expand in various industries and applications.
What is Battery PCB Material ?
The material used to make battery PCBs (Printed Circuit Boards) is typically a type of fiberglass called FR-4, which stands for “Flame Retardant 4.” FR-4 is a composite material made of woven fiberglass cloth and an epoxy resin binder. It is a commonly used material in the electronics industry due to its excellent electrical and mechanical properties.
FR-4 has several advantages that make it ideal for use in battery PCBs. It is a good electrical insulator, meaning that it can prevent electrical current from flowing between the battery and other components. It also has good thermal stability, which means that it can withstand high temperatures without degrading. This is important because batteries can generate a lot of heat during operation.
In addition to FR-4, other materials are also used to make battery PCBs, depending on the application and requirements. For example, flexible PCBs made of polyimide or polyester materials may be used in applications where the battery needs to be flexible or bendable.
Overall, the material used to make a battery PCB plays a crucial role in ensuring the safety and performance of the battery. It is important to choose a material that can withstand the stresses of battery operation and provide good electrical insulation.
Types of Battery PCB
Battery PCBs can be classified into two main categories: construction and performance. The construction category encompasses various designs tailored to meet the specific demands and requirements of customers. Factors such as space requirements, mechanical robustness, stress tolerance, and electrical integrity should be carefully assessed before choosing a PCB. There are several types of battery PCBs available, each with its unique characteristics:
Single-Sided PCB: A type of PCB with circuitry on only one side, commonly used in simpler applications.
Double-Sided PCB: This PCB has circuitry on both sides, allowing for increased circuit complexity and component density.
Rigid PCB: A traditional PCB made from solid materials like fiberglass, providing excellent mechanical stability and strength.
Flexible Circuit Board: As the name suggests, this PCB offers flexibility, enabling it to be bent or molded to fit unconventional or compact spaces.
Multilayer PCB: These PCBs consist of multiple layers of circuitry, interconnected by vias, enabling higher circuit complexity and reduced space requirements.
Rigid-Flex Circuit Board: Combining the advantages of rigid and flexible boards, this PCB offers both mechanical stability and the ability to bend or flex in specific areas.
Based on their performance, battery PCBs can be further categorized into two types:
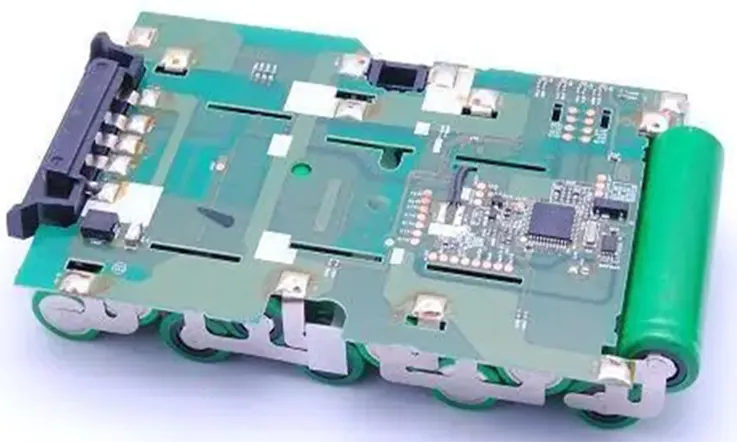
Systems for monitoring and targeting energy use: These systems are designed to monitor energy consumption and identify opportunities for improvement. They provide valuable data and insights for optimizing energy efficiency.
Continuous system commissioning: This category involves customizable systems that can be adjusted according to specific requirements, such as ventilation, heating, air conditioning (HVAC), and other related systems. The aim is to adapt these systems to optimize performance and meet individual needs.
When selecting a battery PCB, it is crucial to consider both the construction aspects, such as space requirements and component durability, as well as the desired performance characteristics, including energy monitoring capabilities or adaptability for system commissioning. This comprehensive evaluation ensures a suitable choice for the intended application
Features of Battery PCB
Battery PCBs provide a comprehensive range of features to ensure the safe and efficient utilization of batteries.
Cell Balancing
Battery PCBs play a crucial role in preserving the overall health and performance of battery packs by effectively managing the charge and discharge cycles of individual cells. By regulating the voltage levels of each cell, these PCBs ensure that they operate within specified ranges. This meticulous control significantly mitigates the potential hazards associated with overcharging and over-discharging, thereby extending the overall lifespan of the battery pack.
Overcharge Protection: Battery PCBs incorporate protective measures against overcharging, which can have severe detrimental effects on batteries. These PCBs are designed to disconnect the power supply when the battery reaches its maximum charge, preventing any further charging.
Over-Discharge Protection: To safeguard batteries from irreversible damage due to excessive discharge, battery PCBs are equipped with over-discharge protection. These PCBs possess the ability to detect when the battery voltage drops below the predetermined threshold, automatically cutting off the power supply to prevent further discharge.
Reverse Polarity Protection
Additionally, battery PCBs offer an important safety feature in the form of reverse polarity protection. This functionality serves to safeguard the battery pack by preventing incorrect connections that could result in a short circuit. By effectively eliminating the risk of reversed polarity, the PCB helps maintain the integrity and safe operation of the battery pack.
Short-Circuit Protection: Short circuits pose a significant risk to batteries, resulting from unintended contact between the positive and negative terminals. Battery PCBs integrate advanced circuitry capable of detecting short circuits promptly. In such cases, the PCBs swiftly disconnect the power supply to shield the battery from potential damage.
High-Quality Base Materials
Battery PCBs are constructed using high-quality materials known for their durability and resistance to environmental factors such as heat and vibrations. This selection of robust materials ensures the long-term reliability and consistent performance of the battery.
Temperature Monitoring
Battery PCBs feature built-in temperature sensors that continuously monitor the battery’s temperature. If the temperature exceeds specified thresholds, indicating excessive heat or extreme cold, the PCBs take preventive action by cutting off the power supply. This prevents further damage to the battery due to adverse temperature conditions.
Voltage Monitoring
Battery PCBs also incorporate voltage monitoring capabilities to effectively monitor the voltage levels of the battery pack. This feature ensures that the voltage remains within a safe operating range, thus enhancing the overall safety and reliability of the battery pack. By continuously monitoring the voltage, the PCB enables prompt action to be taken if the voltage deviates from the desired parameters, helping to prevent potential issues and maintain optimal performance.
Current Limiting
In addition, battery PCBs play a critical role in current regulation within the battery pack. They are responsible for effectively limiting the current flow, thereby preventing overloading and ensuring that the battery does not draw more power than it is capable of handling. This essential function safeguards the battery from excessive stress or potential damage, promoting safe and optimal operation of the battery pack. The PCB’s ability to regulate and restrict current flow contributes significantly to the longevity and safe utilization of the battery pack.
What Are The Drawbacks of Battery PCB ?
Some of the drawbacks of battery PCBs (Printed Circuit Boards) include:
Limited Design Flexibility: Battery PCBs have specific design requirements to accommodate the battery cell’s size, shape, and electrical connections. This limits the overall flexibility of the PCB design, making it challenging to incorporate additional circuitry or optimize the layout.
Higher Cost: Battery PCBs can be more expensive compared to standard PCBs due to the additional components and design considerations required for the battery integration. This can increase the overall production cost of devices utilizing battery PCBs.
Increased Complexity: Integrating batteries into a PCB introduces complexities such as proper power management, charging circuitry, and safety features. These additional circuit elements add complexity to the design, manufacturing, and testing processes.
Space Constraints: Batteries can occupy a significant amount of space on the PCB, limiting the available area for other components. This can be especially problematic in compact electronic devices or where multiple functionalities need to be integrated into a small form factor.
Thermal Management: High-power battery cells can generate heat during operation, requiring proper thermal management considerations. Efficient heat dissipation becomes crucial to prevent overheating and ensure the longevity and safety of the battery and the PCB.
Battery Compatibility: Battery PCBs are built to accommodate specific battery types, sizes, and chemistries. Compatibility issues may arise if a different battery is used, requiring modifications to the PCB design or selection of a different PCB altogether.
Lifespan and Replacement: Batteries have a limited lifespan, typically measured in charge-discharge cycles. When the battery reaches the end of its life, it may need to be replaced. In some cases, replacing the battery in a PCB can be challenging, requiring disassembly and rework.
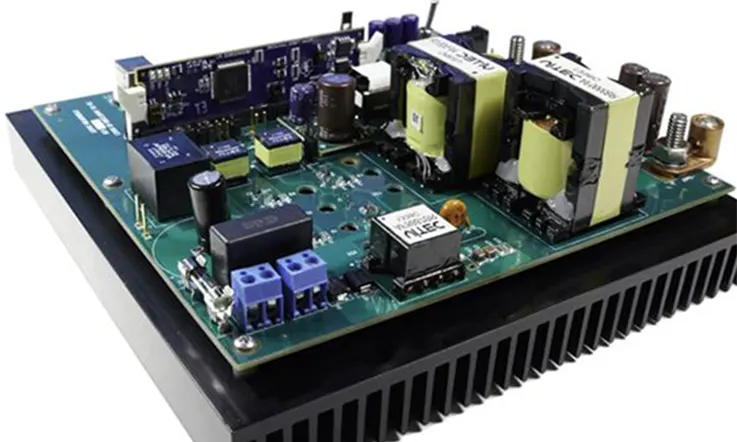
It’s important to note that these drawbacks are specific to integrating batteries into PCBs and may not apply to all applications or designs.
Components of Battery PCBs
The components of a battery PCB can vary depending on the specific type of circuit and the requirements of the battery pack. However, some common components that are typically found in battery PCBs include:
Battery Management System (BMS): This is a key component of a battery PCB that is responsible for monitoring and controlling the battery’s performance. It may include features such as state of charge (SOC) estimation, cell balancing, and temperature monitoring.
Protection Circuit Module (PCM): The PCM is designed to protect the battery from overcharging, over-discharging, and short-circuiting. It typically includes fuses, switches, and other protective devices.
Thermistors: These are temperature-sensitive resistors that are used to monitor the temperature of the battery. They can help to prevent the battery from overheating and can also be used to detect faults in the battery.
Connectors: Battery PCBs may include various types of connectors, such as JST (Japan Solderless Terminal) connectors, to allow for easy connection and disconnection of the battery pack.
Capacitors: Capacitors are used to store energy and can help to stabilize the voltage in the battery pack. They can also help to filter out noise and other disturbances in the circuit.
Resistors: Resistors are used to limit the flow of current in the circuit and can help to protect the battery from overloading or short-circuiting.
To sum up, the components of a battery PCB are designed to work together to provide the necessary protection and monitoring functions for the battery pack. The specific components and their functions will depend on the application and requirements of the battery.
Battery Management System PCB Design
When designing a PCB, it is crucial to incorporate a Battery Management System (BMS), especially for lithium batteries. The BMS plays a vital role in monitoring and controlling the battery’s charging and discharging processes. It consists of several key components, including the main PCB board, protection boards, temperature sensors, current sensors, and other necessary elements.
The primary function of a BMS is to safeguard the lithium battery against potential risks such as overcharging and overheating, thus ensuring its long-term health and optimal performance. Through precise monitoring and control, a well-designed BMS enables safe and efficient charging and discharging operations, ultimately maximizing the lifespan of the battery. Implementing a professionally engineered BMS enhances both the safety and longevity of the entire battery system.
The Importance of Protection Circuit Modules (PCM)
Battery cell manufacturers are gradually shifting from small batteries to larger battery cells, driven by the requirements of critical applications. This trend holds particular significance for industries such as electric car manufacturing, where the need for safe and portable devices is paramount.
Rechargeable lithium batteries necessitate primary and secondary protection mechanisms provided by Protection Circuit Modules (PCMs). These protection mechanisms safeguard the battery from potential risks such as overcharging, overcurrent, over-discharge, and short circuits. As a result, the inclusion of a protection circuit board becomes imperative for any lithium battery.
The protection circuit board, often incorporating a Positive Temperature Coefficient (PTC) component, plays a vital role in ensuring the battery’s safety. This board contains electronic circuits that fulfill the crucial function of protecting the lithium battery. It is essential for the battery core to operate within a specified voltage range, typically designed for environments spanning from -40 degrees to +85 degrees Celsius during the charging and discharging processes.
The principle behind the protection board entails three states: average level, high level, and low level. This principle facilitates the detection of parameters such as overcharge voltage, release voltage of overcharge, detection voltage of over-discharge, release voltage of over-discharge, overcurrent 1 and 2 detection voltage, long short circuit detection voltage, charger detection voltage, normal operation consumption current, and the current consumption during over-discharge.
Ensuring the reliable and comprehensive protection of lithium batteries through well-designed protection circuit boards is crucial in meeting the safety and performance requirements of diverse applications.
Design Considerations for Battery PCBs
When designing a battery PCB, there are several important considerations to take into account to ensure the circuit is safe, reliable, and effective. Some key design considerations for battery PCBs include:
Voltage and Current Ratings: The voltage and current ratings of the battery PCB must be carefully selected to match the requirements of the battery pack and the application. The circuit must be able to handle the maximum voltage and current levels that the battery can produce without damage or failure.
Size and Shape: The size and shape of the battery PCB must be designed to fit within the available space in the battery pack. The circuit should be compact and efficient to minimize the use of space and materials.
Placement of Components: The placement of components on the battery PCB is critical for ensuring proper functionality and safety. Components must be arranged in a way that minimizes the risk of short-circuits and overheating, and allows for efficient heat dissipation.
Heat Dissipation: Overheating can be a major problem in battery PCBs, and proper heat dissipation is essential for preventing damage to the battery and the circuit. The PCB should be designed with sufficient copper traces and vias to allow for effective heat transfer, and may include additional cooling features such as heat sinks or fans.
Environmental Factors: The battery PCB must be designed to withstand the environmental factors that it may be exposed to, such as temperature, humidity, and vibration. The circuit should be protected from moisture and dust, and may include features such as conformal coatings or encapsulation.
Regulatory Compliance: Battery PCBs must comply with various regulatory standards and certifications, such as UL, CE, and RoHS. The design of the circuit must take into account these requirements to ensure that the finished product is safe and compliant.
By taking these design considerations into account, designers can create battery PCBs that are safe, reliable, and effective for a wide range of applications.
Manufacturing Process for Battery PCBs
The manufacturing process for battery PCBs typically involves several stages, including design, prototyping, production, and testing. Here are the general steps involved in the manufacturing process for battery PCBs:
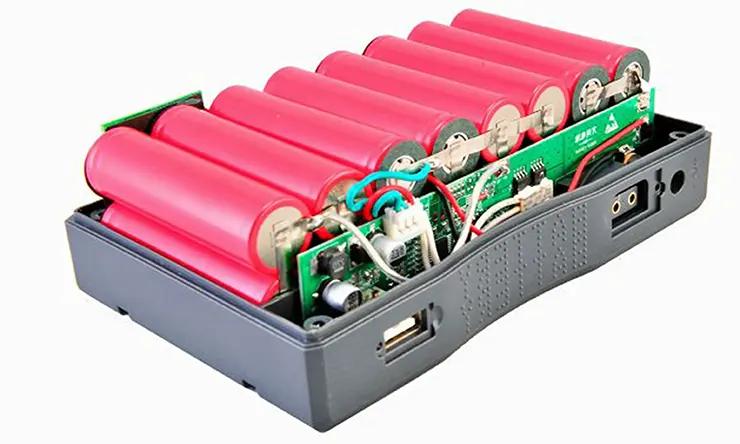
1. Printed Circuit Board (PCB) Design: The first step in the manufacturing process is to design the PCB using specialized software. The design must take into account the requirements of the battery pack, the components that will be used in the circuit, and the manufacturing process itself.
2. Prototyping: Once the PCB design is complete, a prototype is typically created to test the circuit and make any necessary refinements. This can involve printing the circuit design onto a test PCB board and assembling the components onto it.
3. Assembly of Components: After the prototype has been tested and refined, the final production PCB is assembled using automated equipment. This involves placing components onto the PCB board using pick-and-place machines, and then soldering them onto the board using reflow ovens or other soldering equipment.
4. Testing and Quality Control: Once the PCB has been assembled, it is tested to ensure that it meets the required specifications and quality standards. This can involve using automated testing equipment to check for faults, as well as manual inspection by trained technicians.
5. Packaging and Shipping: Once the battery PCB has passed all testing and quality control checks, it is packaged and shipped to the customer or manufacturer of the battery pack.
As a result, the manufacturing process for battery PCBs requires specialized equipment and expertise to ensure that the final product is safe, reliable, and effective. By following these steps, manufacturers can create battery PCBs that meet the required specifications and quality standards for a wide range of applications.
Differences Between a Battery PCB and BMS
In terms of hardware components, protection circuit boards (PCBs) consist of MOS tubes, integrated circuits, and capacitive and resistive elements. On the other hand, a battery management system (BMS) is more advanced, incorporating a microcontroller integrated with intelligent software that functions as the system’s core.
Precision varies between these devices. While a circuit protection module lacks the ability to accurately assess the battery pack’s charging level, a BMS performs calculations, monitors different parameters such as battery charge state and health, and interprets measurements more effectively.
Steering capabilities differ as well. PCBs are unable to control charging or consuming units, while a BMS leverages communication protocols such as MODBUS, I2C, and CAN to steer these units. This steering functionality enables optimization of battery utilization and system behavior, with devices like electric motors being commonly regulated by the BMS.
Regarding low-temperature operation, BMSs exhibit limitations compared to PCBs. They may experience instability under such conditions.
It is important to note that BMSs generally entail a higher cost compared to PCBs, reflecting the advanced features and additional functionalities they offer.
PCB/PCM vs. BMS: Which One Is Better ?
PCB/PCM and BMS are both important components in a battery pack, but they serve different purposes and cannot be directly compared as “better” or “worse.”
A PCB or PCM (Protection Circuit Board/Module) is a small electronic circuit board that is typically integrated into a battery cell to provide protection against overcharging, over-discharging, and short-circuiting. It is a simple and cost-effective solution for protecting individual battery cells, but it cannot monitor or control the overall performance of a battery pack.
On the other hand, a BMS (Battery Management System) is a more advanced electronic system that can monitor and control the performance of an entire battery pack, typically consisting of multiple cells. A BMS can provide functions such as cell balancing, temperature monitoring, and state-of-charge estimation, in addition to protection functions. It is a more complex and expensive solution but provides superior performance and safety for battery packs used in high-end applications.
if you are working with a battery pack consisting of a single cell, a PCB/PCM may be the most appropriate choice for protection. If you are working with a battery pack consisting of multiple cells, a BMS is necessary to ensure optimal performance, safety, and longevity of the battery pack.
Therefore, the suitability of battery protection devices depends on factors such as the battery pack size and the level of protection required. In the case of small battery packs containing 20 cells or less, such as those found in mobile phones, PCBs or PCMs are appropriate. These devices provide essential protection for digital batteries. On the other hand, for large battery packs comprising over 20 cells, BMSs are more suitable as they offer advanced monitoring and precise checks across various aspects of the battery. BMSs are designed to cater to the specific needs of larger battery packs, ensuring comprehensive protection and efficient management.
How To Assemble A Battery PCB?
Assembling a battery PCB (Printed Circuit Board) involves several steps to ensure that the battery operates safely and efficiently. Here are the general steps to assemble a battery PCB:
1. Gather the necessary tools and materials: You will need a soldering iron, solder wire, flux, wire cutters, and the battery PCB.
2. Prepare the battery: Before soldering, ensure that the battery is clean and free of any debris or residue. You can use a clean cloth or alcohol wipe to clean the battery.
3. Solder the battery: Place the battery on the PCB and apply a small amount of flux to the PCB pads. Then, heat the soldering iron and apply it to the pads, adding a small amount of solder to create a secure connection between the battery and the PCB. Be careful not to overheat the battery or the PCB.
4. Solder the components: Once the battery is securely connected, you can begin soldering the other components to the PCB. Follow the manufacturer’s instructions and ensure that the components are correctly positioned and soldered.
5. Inspect and test the PCB: Once all components are soldered, inspect the PCB for any solder bridges or cold solder joints. Use a multimeter to test the voltage and current output of the battery and ensure that it is within the desired range.
6. Assemble the final product: Once the PCB is tested and confirmed to be working correctly, you can assemble it into the final product.
It’s important to note that working with batteries and PCBs can be dangerous, so it’s essential to take proper precautions, such as wearing safety glasses and using proper ventilation. If you are not familiar with soldering or working with electronics, it’s recommended to seek guidance from a professional or experienced hobbyist.
How to Choose a Reliable Battery PCB Manufacturer ?
When considering a PCB manufacturer, several key aspects should be taken into account:
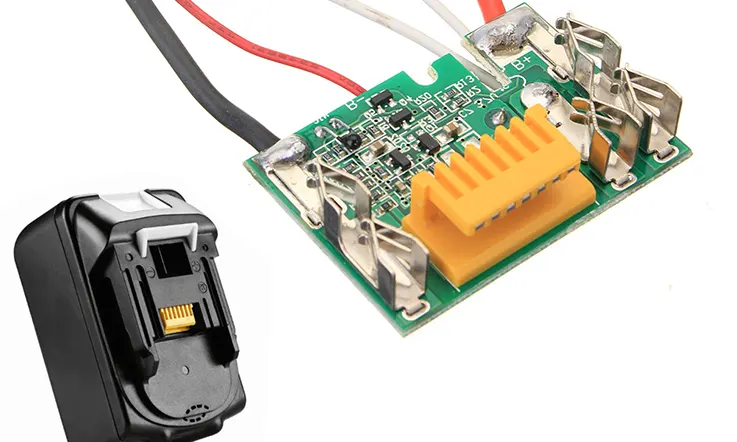
Quality holds paramount importance when selecting a PCB manufacturer. It is crucial to opt for a manufacturer that maintains robust quality control processes to ensure the production of reliable and high-quality PCBs.
Experience in the field is another vital factor to consider. Collaborating with an experienced PCB manufacturer ensures that your product will be manufactured accurately and meets all your specific requirements. Assess the manufacturer’s track record and inquire about their production process to gain confidence in the successful outcome of your project.
Customization capabilities are an essential consideration. Choosing a manufacturer that can customize their Battery PCBs to align with your precise needs and specifications is crucial for achieving optimal results.
Cost is an inevitable factor to consider in the decision-making process. It is recommended to obtain quotes from different manufacturers and compare them carefully to determine the most cost-effective option without compromising quality or customization.
By considering these factors diligently, you can make an informed choice when selecting a PCB manufacturer that will deliver high-quality, customized products at a competitive price point.
Why Choose JarnisTech for Your Battery PCB
JarnisTech is a trusted provider of top-quality Battery PCBs, offering advanced technology and employing the best strategies throughout our manufacturing processes. As your supplier, we are dedicated to meeting your Battery PCB demands effectively. Our expertise lies in customizing Battery PCBs to your precise specifications, ensuring a tailored solution that meets your unique requirements.
At JarnisTech, we prioritize the use of high-quality raw materials in the construction of our Battery PCB products. To enhance durability, reliability, and overall performance, we source our Battery PCBs from reputable international markets. Our commitment to continual improvement drives us to deliver Battery PCBs that optimize efficiency and performance.
We invite you to contact us now and submit your inquiry to experience our exceptional services. Join the ranks of our satisfied customers who have benefited from our reliable and customized Battery PCB solutions.
Conclusion and Future Directions
Battery PCBs play a critical role in protecting and managing rechargeable batteries, ensuring their safety and longevity. Battery PCBs come in different types and configurations, with features such as overcharge and over-discharge protection, balancing, and temperature monitoring. The design and manufacturing of battery PCBs require careful consideration of factors such as voltage and current ratings, size and shape, placement of components, heat dissipation, environmental factors, and regulatory compliance.
Battery PCBs are expected to continue to play a vital role in the development of new technologies, including electric vehicles, renewable energy storage systems, and portable electronics. As battery technology continues to advance, battery PCBs are likely to evolve to meet the growing demands of these applications, with features such as faster charging times, higher power density, and improved safety.
In addition, the trend towards miniaturization and increased functionality is likely to continue, with battery PCBs becoming smaller, more efficient, and integrating additional sensors and monitoring capabilities. Overall, the future of battery PCBs is bright, with the potential to revolutionize the way we store and use energy in a wide range of applications.